Quantum Computing: The Next Big Leap in Technology
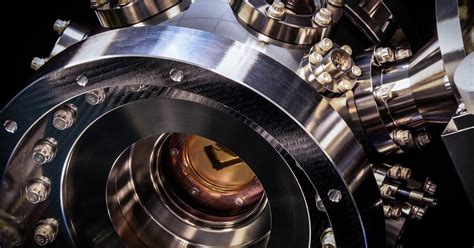
Quantum Computing is poised to revolutionize technology as we know it. This blog post explores the foundations of this cutting-edge field, contrasting quantum computing’s principles with those of classical computing. Unlike traditional bits, quantum bits, or qubits, leverage superposition and entanglement for exponentially greater processing power. Discover the real-world applications of quantum computing, from drug discovery and materials science to financial modeling and cryptography. Finally, we’ll delve into the future trends shaping this exciting domain, while acknowledging the significant challenges that remain in realizing its full potential, including error correction and scalability.Okay, I will create a content section based on your guidelines for the Quantum Computing: The Next Big Leap in Technology blog post. Here’s the content, optimized for SEO and readability, with the specified HTML tags: html
Understanding The Basics Of Quantum Computing Technology
Quantum computing represents a revolutionary approach to computation, leveraging the principles of quantum mechanics to solve complex problems that are beyond the capabilities of classical computers. Unlike classical computers that store information as bits representing 0 or 1, quantum computers use qubits. Qubits can exist in a state of superposition, meaning they can represent 0, 1, or both simultaneously. This capability, along with other quantum phenomena, allows quantum computers to perform calculations in entirely new ways.
At its core, quantum computing harnesses phenomena like superposition and entanglement to perform computations. Superposition allows qubits to exist in multiple states at once, dramatically increasing the computational possibilities. Entanglement, another key principle, links two or more qubits together in such a way that the state of one instantly influences the state of the others, regardless of the distance between them. These properties enable quantum computers to explore a vast number of possibilities simultaneously, making them exceptionally powerful for certain types of calculations.
Key Concepts in Quantum Computing:
- Qubits: The basic unit of quantum information, existing in a superposition of states.
- Superposition: The ability of a qubit to be in multiple states (0 and 1) simultaneously.
- Entanglement: A phenomenon where two or more qubits are linked, and their fates are intertwined.
- Quantum Gates: Operations that manipulate the state of qubits, similar to logic gates in classical computing.
- Quantum Algorithms: Algorithms designed to run on quantum computers, exploiting quantum mechanical effects.
While still in its early stages, quantum computing holds immense promise for transforming various fields. Its potential impact spans drug discovery, materials science, financial modeling, and artificial intelligence. However, building and maintaining quantum computers presents significant technical challenges. Qubits are extremely sensitive to environmental noise, requiring ultra-cold temperatures and precise control to maintain their quantum states. Overcoming these challenges is crucial to realizing the full potential of quantum computing.
How Quantum Computing Differs From Classical Computing
The fundamental distinction between Quantum Computing and classical computing lies in how they process information. Classical computers, which power our everyday devices, store and process information using bits. These bits can represent either a 0 or a 1, a binary state that dictates all operations. Quantum computers, on the other hand, leverage the principles of quantum mechanics to manipulate information in a fundamentally different way. This new way allows them to tackle complex problems that are impossible for even the most powerful supercomputers today.
The implications of this difference are profound, leading to exponential speedups for certain types of calculations. While classical computers perform calculations sequentially, one step at a time, quantum computers can explore multiple possibilities simultaneously. This capability stems from the unique properties of quantum bits, or qubits, which can exist in multiple states at once.
Qubit vs. Bit
The basic unit of information in classical computing is the bit, which can be either 0 or 1. In contrast, a qubit, the basic unit of quantum information, can exist in a state of superposition, representing 0, 1, or both simultaneously. This fundamental difference allows quantum computers to explore a vast number of possibilities concurrently, leading to exponential speedups for certain types of calculations.
Feature | Classical Computing | Quantum Computing |
---|---|---|
Basic Unit | Bit (0 or 1) | Qubit (0, 1, or both) |
Information Storage | Definite State | Superposition |
Processing | Sequential | Parallel |
Computational Power | Limited | Potentially Exponentially Faster |
To further clarify these differences, consider the following points:
- Information Representation: Classical computers use bits to represent data as 0s or 1s.
- Processing Method: They process information sequentially, one step at a time.
- Computational Power: Classical computers are limited by the number of transistors and clock speed.
- Error Correction: They employ error-correcting codes to minimize the impact of errors.
- Problem Solving: Well-suited for linear and straightforward computational tasks.
Superposition and Entanglement
Superposition is the ability of a qubit to exist in multiple states (0 and 1) simultaneously until measured. Entanglement, another key quantum phenomenon, links two or more qubits together in such a way that the state of one instantly influences the state of the other, regardless of the distance separating them. These two principles create the foundation for quantum computers to perform complex calculations in ways that are fundamentally impossible for classical computers.
These properties enable quantum computers to perform certain calculations much faster than classical computers. This quantum advantage has the potential to revolutionize fields such as drug discovery, materials science, and cryptography.
Understanding the distinctions between classical and Quantum Computing highlights the revolutionary potential of quantum technology. While still in its early stages, the development of quantum computers promises to transform industries and solve problems previously considered intractable.
Real-World Applications And Use Cases Of Quantum Computing
Quantum computing, while still in its nascent stages, holds immense potential to revolutionize various industries. Unlike classical computers that store information as bits representing 0 or 1, quantum computers use quantum bits or qubits. Qubits can exist in multiple states simultaneously due to the principles of superposition and entanglement, allowing quantum computers to perform complex calculations at speeds far exceeding those of classical computers. This capability unlocks a wide array of applications that are currently infeasible with existing technology.
One of the most promising areas is optimization. Many real-world problems, such as logistics, supply chain management, and financial portfolio optimization, involve finding the best solution from a vast number of possibilities. Quantum algorithms like the Quantum Approximate Optimization Algorithm (QAOA) are specifically designed to tackle these types of optimization problems, offering the potential for significant improvements in efficiency and cost savings.
Industry | Application | Potential Benefit |
---|---|---|
Healthcare | Drug Discovery | Faster identification of drug candidates |
Finance | Portfolio Optimization | Higher returns with reduced risk |
Logistics | Route Optimization | Reduced transportation costs and delivery times |
Materials Science | Materials Design | Development of novel materials with enhanced properties |
Another significant application lies in the realm of cryptography. Current encryption methods rely on the computational difficulty of certain mathematical problems. However, quantum computers, leveraging algorithms like Shor’s algorithm, pose a threat to these encryption schemes. This has spurred research into quantum-resistant cryptography to secure sensitive data in the face of future quantum attacks.
Drug Discovery
The process of discovering new drugs is often long, expensive, and fraught with uncertainty. Quantum computing offers the potential to accelerate this process by simulating the behavior of molecules and their interactions with drug candidates. This can help researchers identify promising compounds more quickly and efficiently, reducing the time and cost associated with drug development.
Financial Modeling
Financial markets are inherently complex and involve a multitude of variables. Quantum computing can be used to develop more sophisticated financial models that can better predict market trends, optimize investment portfolios, and manage risk. Quantum algorithms can also be used to detect fraud and improve the efficiency of financial transactions.
- Steps to Implement Quantum Algorithms:
- Identify a suitable problem that benefits from quantum computation.
- Develop or adapt a quantum algorithm to address the specific problem.
- Simulate the quantum algorithm on classical computers for testing and validation.
- Translate the quantum algorithm into a format suitable for execution on quantum hardware.
- Run the algorithm on a quantum computer.
- Analyze the results obtained from the quantum computer.
- Iterate and refine the algorithm based on the results.
Materials Science
The discovery and design of new materials with specific properties is crucial for advancements in various fields, including energy, electronics, and medicine. Quantum computing can be used to simulate the behavior of atoms and molecules, allowing researchers to design materials with desired characteristics, such as superconductivity or enhanced strength. This can lead to the development of novel materials with transformative applications.
As quantum technology matures, we can expect to see even more innovative applications emerge. However, significant challenges remain, including the development of more stable and scalable qubits, as well as the creation of quantum algorithms that can outperform classical algorithms for a wider range of problems. Despite these challenges, the potential benefits of quantum computing are so significant that it is likely to remain a major area of research and development for years to come.
Here’s the content section for your article:
Future Trends And The Challenges In Quantum Computing
The future of quantum computing is brimming with potential, poised to revolutionize numerous sectors. As the technology matures, we can anticipate quantum computers becoming more accessible and integrated into mainstream computing infrastructure. Cloud-based quantum services will likely play a crucial role, allowing researchers and businesses to leverage quantum resources without the need for expensive hardware investments. Moreover, hybrid quantum-classical algorithms will become more sophisticated, enabling us to solve increasingly complex problems that are currently intractable.
Year | Market Size (USD Billion) | Key Advancements |
---|---|---|
2024 | 10.4 | Improved qubit stability, enhanced error correction |
2027 | 34.5 | Wider availability of quantum cloud services |
2030 | 86.0 | Integration with classical high-performance computing |
2033 | 190.0 | Quantum computers solving real-world industrial problems |
Despite the optimistic outlook, significant challenges remain. Building and maintaining stable qubits is a formidable task, as they are extremely susceptible to environmental noise, leading to decoherence. Error correction in quantum computers is another critical hurdle, requiring innovative techniques to mitigate the effects of noise and ensure accurate computations. Furthermore, the development of quantum algorithms is still in its early stages, and there is a need for more quantum software and programming tools that are accessible to a wider range of developers. The skills gap in quantum computing is also a concern, highlighting the importance of education and training programs to prepare the workforce for the quantum era.
Next Steps for Exploring Quantum Computing:
- Enroll in online courses or workshops focused on quantum computing fundamentals.
- Familiarize yourself with quantum programming languages such as Qiskit or Cirq.
- Explore open-source quantum software libraries and contribute to community projects.
- Read research papers and articles to stay updated on the latest advancements.
- Attend quantum computing conferences and networking events.
- Consider pursuing a degree or specialization in quantum information science.
Addressing these challenges will require concerted efforts from researchers, industry experts, and policymakers. Investment in research and development is crucial to drive breakthroughs in qubit technology, error correction, and quantum algorithm design. Collaboration between academia and industry can accelerate the translation of research findings into practical applications. Moreover, fostering a supportive ecosystem for quantum startups and entrepreneurs can spur innovation and accelerate the commercialization of quantum technologies. Overcoming these hurdles will pave the way for quantum computing to realize its full potential and transform industries across the board.
The coming decade will be pivotal for quantum computing. Success hinges on addressing the core challenges of qubit stability and scalability, while simultaneously fostering a robust ecosystem of quantum software and talent. – Dr. Eleanor Shaw, Quantum Computing Researcher